ALPHA-, BETA- AND GAMMA-RAY SPECTROSCOPY - INTRODUCTION
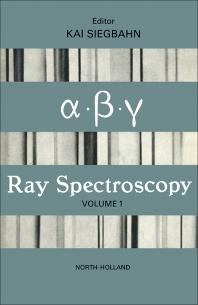
Our knowledge concerning nuclear structure and nuclear forces comes from three main sources: (1) radioactive decay, (2) nuclear reactions and (3) high energy or elementary particle reactions. There is a certain overlap, particularly with the first two of these, both with regard to the experimental techniques and to the type of information which is obtained. The third one is gradually drifting away from the other two and becoming a study of a new physical domain, in some respects equally distant from low energy nuclear physics as this is from atomic shell physics.
Alpha-, beta- and gamma-ray spectroscopy is often used synonymously to the first mentioned field, although these radiations, of course, also occur in nuclear reactions. In radioactive decay only the lowest nuclear levels are excited, up to some few MeV. It is important to bear this in mind because of the inherent limitations to the information which result from it. The phenomena which are accessible for study at these energies are, however, of basic importance for the understanding of nuclear structure in general. Furthermore, they are not masked by the increasing complexity occurring at higher excitations with gradual deterioration into statistical processes.
The transition probabilities in /?-decay are governed by selection rules and can be determined experimentally by measuring the partial half-lives of the different branches of the decay. This can be accomplished by means of the so-called Fermi analysis. One can then calculate the//-values of the transitions, / being the partial half-life and / a function of the transition energy and the atomic charge. The//-value is proportional to the inverse square of the nuclear matrix element. The //-values of various ^-transitions can be grouped into different classes. Using this classification and the particular selection rules which apply to the different classes, one can draw conclusions regarding the changes in spin, parity and orbital angular momentum associated with the transitions.
As mentioned above, a fundamental problem with special bearing upon the nature of nuclear forces is the form of the Hamiltonian describing the interaction between heavy and light particles in ^-decay. This problem was previously mostly studied by the shapes of the continuous spectra of forbidden transitions.
The form of the interaction term in yff-decay can also be studied by means of the angular correlation between electron and neutrino. In this case also, allowed transitions in principle yield the desired information. The low energies of the recoiling atoms (of the order of 100 eV) strongly favour the use of radioactive gases in such experiments. Only a few have been performed, but they show, among other things, that a continuous neutrino spectrum is emitted during/?-decay, whereas monoenergetic neutrinos are emitted in K-capture. There are five possible types of relativistically invariant interactions in /?-decay, described by a scalar (S), vector (V), tensor (T), axial vector (A) and a pseudoscalar (P), respectively. Experiments must determine the correct combination of the different invariants. The possible invariants do not all yield the same selection rules. Thus the scalar and vector interactions follow the so-called Fermi selection rules, stating that, for allowed transitions, the spin change is zero and the parity is unchanged. With the tensor and axial vector interactions the Gamow-Teller selection rules apply, in which case the spin change is either zero or one for an allowed transition, the parity again being unchanged. There is definite experimental evidence for both types of inter actions. By comparing, for example, the empirically found //-values of superallowed ^-transitions occurring between mirror nuclei having closed shells plus or minus one nucléon, for which the matrix elements can be well estimated by means of single- particle wave functions, it can also be concluded that the Feimi and Gamow-Teller couplings give about equal contributions. Other evidence supports this result.
Some years ago experimental evidence seemed to favour a combination of a scalar and a tensor interaction in /?-decay. A new neutrino recoil experiment, however, gave a conflicting result and the confused situation was carefully reexamined. It was then found that the combination V-A was the only one which could fit the results of all the new experiments. Final and decisive evidence was delivered by an ingenious nuclear resonance scattering experiment performed on circularly polarized y-radiation from Eu . This experiment formed one of the high-lights of the 'parity era' starting with the discovery in 1957 that parity, contrary to all previous concepts, was not conserved in weak inter6ac0tions such as /?-decay. This was proved by the famous experiment on aligned Co . It was found that the electrons were preferentially emitted in one direction, namely opposite to the nuclear spin. Since nuclear spin is an axial vector and the linear momentum of an electron is a polar vector, the parity operation, i.e. a space reflexion, changes the system. There are several consequences of this phenomenon. Decay electrons from an unoriented source are emitted longi tudinally polarized: negative electrons with 'left-handed' spins (negative 'helicity'), i.e. opposite to its motion, and positrons with righ1t-h5man2ded spins (positive helicity). The nuclear resonance scattering experiment on E u showed, incidentally, that the neutrino is a left-handed particle and that the antineutrino is a right-handed one. These circumstances form the basis for the so-called two-component neutrino theory. The polarization of electrons can be found by turning the spin axis through 90° in an external field with a subsequent scattering, showing left-right asymmetry. Another possibility is to observe the circular polarization of the bremsstrahlung produced when the electrons are absorbed.