Appendix 8: The Articulation of Theory: Weak Interactions
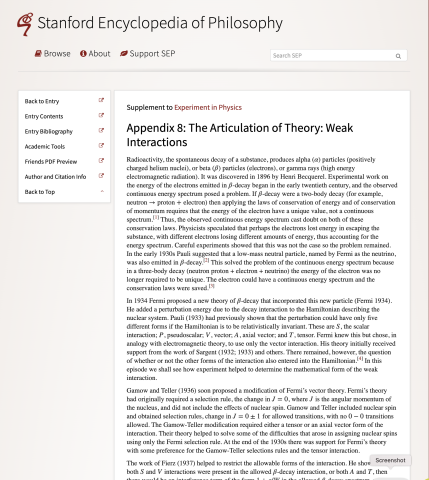
Radioactivity, the spontaneous decay of a substance, produces alpha (αα) particles (positively charged helium nuclei), or beta (ββ) particles (electrons), or gamma rays (high energy electromagnetic radiation). It was discovered in 1896 by Henri Becquerel. Experimental work on the energy of the electrons emitted in ββ-decay began in the early twentieth century, and the observed continuous energy spectrum posed a problem. If ββ-decay were a two-body decay (for example, neutron →→ proton ++ electron) then applying the laws of conservation of energy and of conservation of momentum requires that the energy of the electron have a unique value, not a continuous spectrum.[1] Thus, the observed continuous energy spectrum cast doubt on both of these conservation laws. Physicists speculated that perhaps the electrons lost energy in escaping the substance, with different electrons losing different amounts of energy, thus accounting for the energy spectrum. Careful experiments showed that this was not the case so the problem remained. In the early 1930s Pauli suggested that a low-mass neutral particle, named by Fermi as the neutrino, was also emitted in ββ-decay.[2] This solved the problem of the continuous energy spectrum because in a three-body decay (neutron proton + electron + neutrino) the energy of the electron was no longer required to be unique. The electron could have a continuous energy spectrum and the conservation laws were saved.[3]
The most important of the experiments performed at this time was the measurement of the angular correlation in the decay of He6He6. This decay was a pure Gamow-Teller transition and thus was sensitive to the amounts of AA and TT present in the decay interaction. The decisive experiment was that of Rustad and Ruby (1953; 1955). This experiment was regarded as establishing that the Gamow-Teller part of the interaction was predominantly tensor. This was the conclusion reached in several review papers on the nature of ββ-decay. (Ridley 1954; Kofoed-Hansen 1955; Wu 1955). The experimental apparatus is shown in Figure 17. The definition of the decay volume was extremely important. In order to measure the angular correlation one must know the position of the decay so that one can measure the angle between the electron and the recoil nucleus. The decay volume for the helium gas in this experiment was defined by a 180 microgram/cm2 aluminum hemisphere and the pumping diaphragm. Rustad and Ruby (1953) presented two experimental results. The first was the coincidence rate as a function of the angle between the electron and the recoil nucleus for electrons in the energy range (2.5–4.0) mc2. The second was the energy spectrum of the decay electrons with the angle between the electron and the recoil nucleus fixed at 180°. Both results are shown in Figure 18 along with the predicted results for AA and TT, respectively. The dominance of the tensor interaction is clear. This conclusion was made more emphatic in their 1955 paper which included more details of the experiment and even more data. The later results, shown in Figure 19, clearly demonstrate the superior fit of the tensor interaction.